Stephen Foster and John Chilton* examine the links between groundwater and global change, food, ecosystems, cities, health and energy generation.
Groundwater is critical to the achievement of the new UN-Sustainable Development Goals (SDGs) for 2030, with linkages to global change, food security, ecosystem conservation, resilient cities, human health and energy generation. These linkages are fully explored in a related Strategic Overview Series recently released by the IAH (International Association of Hydrogeologists), in support of global efforts in respect of the UN-SDGs, and marking the IAH’s 60th birthday.
Picture: Urban tubewell, designated arsenic free (painted green) in Khulna, Bangladesh.
Groundwater is a key natural resource, supporting human well-being and economic development – yet it is still quite widely misunderstood, undervalued, poorly managed and inadequately protected. Anthropogenic perturbation of groundwater systems accelerated markedly during the 20th Century as a result of massive exploitation - for urban water-supply and irrigated agriculture - and of radical land-use changes in many aquifer-recharge zones.
Worldwide, intensive exploitation of groundwater resources followed major advances in geological knowledge, waterwell drilling, pump technology and rural electrification, with rapid expansion from the 1950s onwards. Comprehensive statistics on groundwater abstraction and use are (sadly) not available, but global withdrawals are estimated to have reached 900km3 per year in 2010 – providing some 36% of potable water-supply, 42% of water for irrigated agriculture and 24% of direct industrial water-supply.
Diagram: Origins and mechanisms of groundwater salinization.
This has caused growing concerns over sustainability, quality degradation and impacts on dependent ecosystems. Moreover, despite notable technological advances, it is still not straightforward to provide a quantitative assessment of groundwater resource and quality status globally, given the generally inadequate investment in monitoring, and frequent technical difficulty with aggregation of monitoring results.
Global change & groundwater
Groundwater resources stored in aquifers represent the planet’s major reserve of fresh water and they provide both urban and rural communities with an important buffer against drought. This buffer becomes even more essential if global warming gives rise to prolonged or repeated droughts over several consecutive years. But questions then arise as to how intrinsically resilient groundwater reserves themselves are to climate change, and whether we are doing enough to conserve and protect them.
Diagram: Cumulative depletion of groundwater storage reserves, mainly by agricultural irrigation (Konikow 2011)
Groundwater systems (or aquifers) are widely distributed and their replenishment occurs naturally in aquifer-recharge zones, as a result of infiltration of excess rainfall, from bodies of surface-water, and from some human activities – such as infiltration from agricultural irrigation and seepage from urban water and sanitation infrastructure. Prior to large-scale anthropogenic activity (pre-1850 in some places but mainly pre-1950), our capacity to modify, abstract and pollute groundwater regimes was tiny in comparison to the available resource. Most groundwater systems (outside hyper-arid regions) were in an equilibrium state between recharge and discharge, and natural groundwater quality was for the most part excellent.
Significant uncertainty remains over what precise effect the current global warming trend will have on groundwater recharge in different regions. On the one hand, in many humid regions higher ambient temperatures will be accompanied by more frequent high-intensity rainfall and increased rates of groundwater recharge for the same annual rainfall. This is so because a lot of deep infiltration is associated with exceptional rainfall episodes, and in some settings can cause so-called ‘groundwater floods’.
On the other hand, higher temperatures will deplete soil-moisture: increased aridity then leads to soil erosion and gullying, or to soil compaction, which would reduce infiltration capacity. And it is important to note that the ‘natural rates’ of climate and land-cover change regularly experienced over the past 400,000 years were very much slower than those of recent human-induced change. The lowest predicted rate of current global warming is about 10 times greater than anything that has occurred previously. This gives rise to considerable concern about its impact on groundwater recharge, particularly in semi-arid regions.
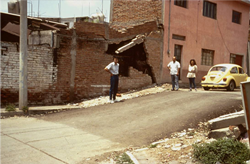
Picture: Major subsidence in major Mexican town resulting from excessive water-well extraction.
But notwithstanding these complexities global warming will, eventually, impact groundwater resources. Graphic evidence of this exists in the palaeo-environmental record gleaned from the study of large aquifers in today’s arid regions. These regions contain groundwater at depth that can be 5000 to 200,000 years old, and which originated as recharge in past millennia that were wetter and colder than the present, with little rainfall recharge (<5mm per annum) taking place subsequently.
Since current groundwater recharge is responsible for (at most) only a tiny fraction of groundwater stored in such aquifer systems, these resources can be regarded as completely resilient to current climatic variability, but are also, in effect, ‘non-renewable’. As such their use (which currently is more than 100 km3 per annum) will be time-limited and contribute to the global transfer of water from the land to the oceans (thus contributing to sea-level rise).
Given the storage inertia of all other aquifer systems, only sustained climate change will seriously deplete available groundwater reserves. But in contrast, increased global demands for groundwater abstraction - and some types of major land-use change - are capable of exerting a marked impact on both recharge and quality of groundwater within decades. Thus, looking forward, we will need to manage the combined impacts of human-induced global warming, land-use change and groundwater exploitation together. More broadly, in taking stock of global and national trends in environmental capital, it will be essential to include full consideration of anthropogenic depletion and degradation of groundwater reserves.
Food security
Picture: A Saharan oasis – formed by discharge of groundwater storeg in alarge aquifer system for 10,000 – 100,000 years.
Groundwater was a critical factor in the green revolution’ (1970-2000), which increased food production by 250% over that period. Private water-well construction for agricultural irrigation has seen remarkable levels of investment, because groundwater supplies are more reliable than surface water and can produce higher crop-yields and economic returns. While groundwater storage is very large, current withdrawal-rates for irrigated agriculture in more arid areas are not physically sustainable, resulting in the long-term (semi-permanent) depletion of aquifer reserves. Elsewhere, widespread waterlogging and salinization of soil and shallow groundwater is an insidious menace, resulting from inadequate management of irrigation water, often in large surface-water distribution systems. The implication of both of these threats is that at least 15% (and perhaps considerably more) of current global food production is not sustainable in the long term.
Additionally, agricultural land-use practices affect groundwater recharge rates and quality, with intensification of cropping leading to diffuse pollution of groundwater by plant nutrients, salinity and some pesticides. Improved land management measures need to be promoted, to provide farmers with incentives to enhance groundwater recharge rates and reduce agrochemical leaching.
Overall, we need to mobilise groundwater professionals, water-resource managers and irrigation engineers to identify governance and management responses to improve resource sustainability with:
- sustainable irrigation and groundwater management plans for aquifers under pressure from irrigated agriculture
- conjunctive management of groundwater and surface-water in major alluvial areas to enhance agricultural productivity and avoid land-drainage problems
- re-alignment of government financing of agriculture (such as crop guarantee prices and pumping-energy subsidies) to reflect limited groundwater availability
- careful evaluation and monitoring to ensure that crop irrigation practices and new irrigation developments do not lead to salinisation.
Ecosystem conservation
Groundwater-dependent ecosystems (GDEs) comprise a complex subset of ecosystems of major significance in the conservation of biodiversity - including many vital sites covered by the RAMSAR Convention (an international treaty for the conservation and sustainable use of wetlands, signed in 1971). GDEs have direct value for the human population, from fish and plant production, water storage and purification, and (indirectly) in terms of landscape and habitat. There is a pressing need to identify GDEs according to type – aquatic, terrestrial, subterranean – and to improve understanding of how they relate to the physical and chemical status of groundwater.
Picture: Indian farmers measuring groundwater levels to inform dry-season cropping plan.
Degradation of GDEs can occur because of anthropogenic modification to aquifer flow-regimes, and the salinisation or pollution of their groundwater. Potentially negative ecological impacts, with the extermination of key species, can arise from uncontrolled groundwater withdrawals for irrigated agriculture or urban water-supply and/or modest increases in groundwater salinity and/or pollution (with nutrients and pesticides).
Social awareness of the importance of groundwater for sustaining viable ecosystems must be promoted to mobilise appropriate stakeholders for GDEs (such as conservation NGOs and local land authorities). GDEs can be conserved by integrating their protection into basin and aquifer-scale water-resource planning and management, or at least acting selectively to Incorporate GDE protection zones into overall groundwater resource use and land-use control policy. In certain cases, augmenting groundwater inflows to aquatic ecosystems can be considered if the water-table should fall below a defined critical level.
Resilient cities
Groundwater is a major source of urban water-supply worldwide, and aquifer storage represents a key resource for urban water-supply security under climate change and extended drought. To achieve this, however, groundwater must be managed more effectively through promoting, as ‘best engineering practice’:
- the establishment of more water-utility wellfields outside cities, and declaring their ‘capture areas’ as drinking-water protection zones
- the more widespread use of groundwater and surface-water resources conjunctively
- the adoption of ‘adaptive management strategies’, recognising that aquifers are in continuous evolution, with some uncertainty over their precise behaviour.
Private water-well construction for in situ self-supply has ‘mushroomed’ in most developing cities, as a ‘coping strategy’ during periods when the water utilities fail. Such water-well use is then often continued for years afterwards as a ‘cost-reduction strategy’. Such (largely unregulated) private wells often draw their water from shallow aquifers that have already been polluted by local urban wastewater or industrial activity. Broad groundwater quantity, quality and economic assessments of current and likely private waterwell use need to be undertaken to allow the public administration to formulate a balanced urban water policy.
Diagram: Complex relationship between urban subsurface infrastructure and shallow groundwater flow.
Moreover, in the developing w
orld, in situ sanitation presents a related hazard to groundwater quality in shallow aquifers. With urban sanitation still lagging far behind water-service provision, the UN-SDGs will (hopefully) see greater emphasis on closing this gap. The sanitary engineering effort required will need to be proactively managed on an integrated basis with groundwater considerations.
Urbanisation greatly modifies the ‘groundwater cycle’ – with marked impacts both during periods of declining aquifer pressures (causing land subsidence with building and infrastructure damage) and of rising water-table (leading to groundwater flooding, with public health hazards and infrastructure damage). Groundwater is often the ‘invisible link’ between various facets of the urban infrastructure, tending to affect ‘everybody’ - while all too often being the responsibility of ‘nobody’.
A more integrated approach is universally required to reduce cost and improve resilience of the water infrastructure. This will necessitate establishing ‘cross-sector urban groundwater consortia’ of all major stakeholders and regulatory agencies, informed by adequate groundwater monitoring, and empowered to implement management plans.
Human health
The naturally excellent quality of most groundwaters, resulting from the self-purification capacity of subsurface strata, has long been a vital factor for human health and well-being. But a prerequisite for preserving this quality is that potable groundwater sources be carefully sealed, to prevent the direct entry of pollutants (such as pathogenic organisms and hydrocarbon fuels or lubricants) from the land surface. All water-wells and springs used as sources of drinking-water require quality surveillance in relation to perceived pollution or contamination risks. If used untreated, wells at serious risk (or already impacted) should be clearly marked as suitable only for non-drinking use.
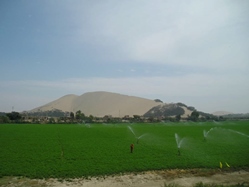
Picture: Groundwater being used to irrigate export asparagus in southern Peru
Aquifers exploited for drinking-water should be subject to systematic assessment of both actual pollution and potential vulnerability from pathogenic microorganisms (acute health hazard) or chemical pollutants (chronic health risk). The situation can then be managed by designating carefully-invigilated land surface protection zones, of dimensions appropriate to contaminant travel in the local hydrogeological conditions and in which potentially-polluting activities can be controlled.
The groundwater of some aquifer systems (such as shallow sands and gravels, and karstic limestones) is rapidly affected by conditions at surface and thus especially vulnerable to pollution from a wide range of contaminants (including the protozoan Cryptosporidium and the cholera bacterium Vibrio cholerae), and so requires additional protection measures.
The most widely-distributed threat to potable groundwater quality comes from land-cultivation for intensive agriculture, which employs nutrients and pesticides that leach from soils to underlying aquifers. Some synthetic organic chemicals (including the so-called ‘emerging contaminants’ with endocrine-disrupting or carcinogenic implications) are resistant to degradation in the subsurface and constitute a long-term health hazard.
However, currently the most serious groundwater contamination and health issues in terms of global population directly impacted are ‘geogenic’ – relating to excessive arsenic and fluoride concentrations arising naturally in some areas through dissolution of sediment or rock under certain hydrochemical conditions, and where rural dwellers take their domestic water-supply directly from village or private water-wells.
Energy generation
Picture: Domestic water-supply collection in Asia from protected springhead.
Onshore hydrocarbon exploitation of all types requires full hydrogeological risk assessment, appropriate environmental regulation, diligent operational control and secure management of subsurface waste injection. In non-conventional hydrocarbon development the principal concern is to prevent shallow aquifer pollution with formation brines, hydrocarbon compounds, fracking fluids and ‘stray gas’. Better cooperation and research are required to monitor and evaluate the groundwater impacts of shale-gas fracking. Applied hydrogeological science makes a pivotal contribution to the development of alternative non-carbon energy sources such as :
- hydrogeothermal energy (particularly of ‘very low enthalpy’ for space cooling or heating) – for which long-term monitoring and modelling of groundwater system response is required to assess sustainability and improve design
- nuclear power – for power-station siting and radioactive waste disposal, as part of the technical platform required to build political and public confidence in the selection of low-risk sites and of safe geological repositories for radioactive waste.
Globally, groundwater pumping for irrigated agriculture is a major energy consumer. Much more effort needs to be put into reducing inefficient energy use due to aquifer depletion and inappropriate well design. Conversely, promoting solar-panels for powering private, unregulated irrigation water-wells in more arid terrains could put groundwater resources under heavier pressure, unless an adequate incentive for ‘grid buy-back’ is introduced in tandem. And, for municipal groundwater sources, effective land management to ensure quality protection would avoid the large energy cost of treatment (to remove pollutants such as nitrates, pesticides and industrial chemicals).
Conclusion
We have endeavoured to highlight the main linkages between society and groundwater, in terms of the aspirations for global sustainable development recently articulated in the UN-SDGs for 2030. Groundwater resources will underpin several SDGs (including SDG-6 for Water Services, SDG-2 for Food Security, SDG-11 for Resilient Cities & SDG-15 for Ecosystem Conservation), and their continuing physical depletion and quality degradation have to be of major concern.
Further reading
The full texts of the IAH Strategic Overview Series on: Global Change, Food Security, Ecosystem Conservation, Resilient Cities, Human Health, Energy Generation & Groundwater can be accessed at https://iah.org/knowledge/learning-resources. Readers interested in the full range of IAH member services should approach the IAH British National Chapter (http://www.iah-british.org/), which is a ‘sister organisation’ to the Geological Society of London Hydrogeology Group.
Picture: Covers for the IAH leaflet series.
* Prof Stephen Foster (Corresponding author E: DrStephenFoster@aol.com) is Global Water Partnership Senior Adviser (www.gwp.org) publishing on trans-sectoral aspects of groundwater management. Dr John Chilton, Executive Manager, IAH, and formerly chair of the British chapter of IAH and Associate Editor of Hydrogeology Journal.